Abstract
Chemical reactions tend to be conceptualized in terms of individual molecules transforming into products, but are usually observed in experiments that probe the average behaviour of the ensemble. Single-molecule methods move beyond ensemble averages and reveal the statistical distribution of reaction positions, pathways and dynamics1,2,3. This has been shown with optical traps and scanning probe microscopy manipulating and observing individual reactions at defined locations with high spatial resolution4,5, and with modern optical methods using ultrasensitive photodetectors3,6,7 that enable high-throughput single-molecule measurements. However, effective probing of single-molecule solution chemistry remains challenging. Here we demonstrate optical imaging of single-molecule electrochemical reactions7 in aqueous solution and its use for super-resolution microscopy. The method utilizes a chemiluminescent reaction involving a ruthenium complex electrochemically generated at an electrode8, which ensures minimal background signal. This allows us to directly capture single photons of the electrochemiluminescence of individual reactions, and to develop super-resolved electrochemiluminescence microscopy for imaging the adhesion dynamics of live cells with high spatiotemporal resolution. We anticipate that our method will advance the fundamental understanding of electrochemical reactions and prove useful for bioassays and cell-imaging applications.
Access options
Subscribe to Journal
Get full journal access for 1 year
199,00 €
only 3,90 € per issue
Tax calculation will be finalised during checkout.
Rent or Buy article
Get time limited or full article access on ReadCube.
from$8.99
All prices are NET prices.
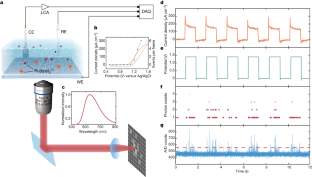
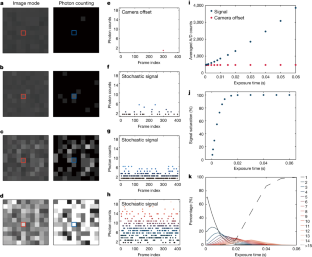
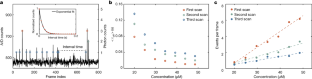
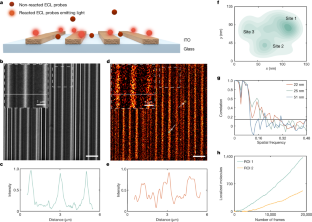
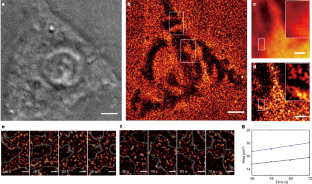
Data availability
The data that support the findings of this study are included in the paper and its Supplementary Information and Supplementary Videos and are available from the corresponding author upon reasonable request.
References
- 1.
Ho, W. Single-molecule chemistry. J. Chem. Phys. 117, 11033–11061 (2002).
- 2.
Roeffaers, M. B. J. et al. Spatially resolved observation of crystal-face-dependent catalysis by single turnover counting. Nature 439, 572–575 (2006).
- 3.
Sambur, J. B. et al. Sub-particle reaction and photocurrent mapping to optimize catalyst-modified photoanodes. Nature 530, 77–80 (2016).
- 4.
Liu, L. R. et al. Building one molecule from a reservoir of two atoms. Science 360, 900–903 (2018).
- 5.
de Oteyza, D. G. et al. Direct imaging of covalent bond structure in single-molecule chemical reactions. Science 340, 1434–1437 (2013).
- 6.
Chen, T. et al. Optical super-resolution imaging of surface reactions. Chem. Rev. 117, 7510–7537 (2017).
- 7.
Collinson, M. M. & Wightman, R. M. Observation of individual chemical reactions in solution. Science 268, 1883–1885 (1995).
- 8.
Bard, A. J. Electrogenerated Chemiluminescence (CRC Press, 2004).
- 9.
Zhang, J., Arbault, S., Sojic, N. & Jiang, D. Electrochemiluminescence imaging for bioanalysis. Annu. Rev. Anal. Chem. 12, 275–295 (2019).
- 10.
Miao, W. J., Choi, J. P. & Bard, A. J. Electrogenerated chemiluminescence 69: the tris(2,2'-bipyridine)ruthenium(II), (Ru(bpy)32+)/tri-n-propylamine (TPrA) system revisited—a new route involving TPrA·+ cation radicals. J. Am. Chem. Soc. 124, 14478–14485 (2002).
- 11.
Sentic, M. et al. Mapping electrogenerated chemiluminescence reactivity in space: mechanistic insight into model systems used in immunoassays. Chem. Sci. 5, 2568–2572 (2014).
- 12.
Fan, F. R. F. & Bard, A. J. Observing single nanoparticle collisions by electrogenerated chemiluminescence amplification. Nano Lett. 8, 1746–1749 (2008).
- 13.
Ma, C. et al. Dynamically imaging collision electrochemistry of single electrochemiluminescence nano-emitters. Chem. Sci. 9, 6167–6175 (2018).
- 14.
Jin, Z. & Bard, A. J. Atom-by-atom electrodeposition of single isolated cobalt oxide molecules and clusters for studying the oxygen evolution reaction. Proc. Natl Acad. Sci. USA 117, 12651–12656 (2020).
- 15.
Chen, M. M. et al. Spatiotemporal imaging of electrocatalytic activity on single 2D gold nanoplates via electrogenerated chemiluminescence microscopy. Chem. Sci. 10, 4141–4147 (2019).
- 16.
Islam, M. Einstein–Smoluchowski diffusion equation: a discussion. Phys. Scr. 70, 120–125 (2004).
- 17.
Bard, A. J. & Faulkner, L. R. Electrochemical Methods: Fundamentals and Applications (Wiley, 2000).
- 18.
Cid, C. P., Spada, E. & Sartorelli, M. Effect of the cathodic polarization on structural and morphological proprieties of FTO and ITO thin films. Appl. Surf. Sci. 273, 603–606 (2013).
- 19.
Xu, K., Babcock, H. P. & Zhuang, X. Dual-objective STORM reveals three-dimensional filament organization in the actin cytoskeleton. Nat. Methods 9, 185–188 (2012).
- 20.
Voci, S. et al. Surface-confined electrochemiluminescence microscopy of cell membranes. J. Am. Chem. Soc. 140, 14753–14760 (2018).
- 21.
Rust, M. J., Bates, M. & Zhuang, X. W. Sub-diffraction-limit imaging by stochastic optical reconstruction microscopy (STORM). Nat. Methods 3, 793–796 (2006).
- 22.
Sharonov, A. & Hochstrasser, R. M. Wide-field subdiffraction imaging by accumulated binding of diffusing probes. Proc. Natl Acad. Sci. USA 103, 18911–18916 (2006).
- 23.
Chovin, A., Garrigue, P., Vinatier, P. & Sojic, N. Development of an ordered array of optoelectrochemical individually readable sensors with submicrometer dimensions: application to remote electrochemiluminescence imaging. Anal. Chem. 76, 357–364 (2004).
- 24.
Nieuwenhuizen, R. P. J. et al. Measuring image resolution in optical nanoscopy. Nat. Methods 10, 557–562 (2013).
- 25.
Deschout, H. et al. Precisely and accurately localizing single emitters in fluorescence microscopy. Nat. Methods 11, 253–266 (2014).
- 26.
Innocenzi, P., Kozuka, H. & Yoko, T. Fluorescence properties of the Ru(bpy)32+ complex incorporated in sol−gel-derived silica coating films. J. Phys. Chem. B 101, 2285–2291 (1997).
- 27.
Geiger, B., Spatz, J. P. & Bershadsky, A. D. Environmental sensing through focal adhesions. Nat. Rev. Mol. Cell Biol. 10, 21–33 (2009).
- 28.
Deschout, H. et al. Complementarity of PALM and SOFI for super-resolution live-cell imaging of focal adhesions. Nat. Commun. 7, 13693 (2016).
- 29.
Ding, H., Guo, W. & Su, B. Imaging cell‐matrix adhesions and collective migration of living cells by electrochemiluminescence microscopy. Angew. Chem. Int. Ed. 132, 457–464 (2020).
- 30.
Valenti, G. et al. Single cell electrochemiluminescence imaging: from the proof-of-concept to disposable device-based analysis. J. Am. Chem. Soc. 139, 16830–16837 (2017).
Acknowledgements
This work was funded by the National Natural Science Foundation of China (21974123), the Natural Science Foundation of Zhejiang Province (LR20B050002), the Fundamental Research Funds for the Central Universities (2019XZZX003-01) and the Hundreds Program of Zhejiang University. We thank G. Tang for advice with cell culture, the Micro and Nano Fabrication Centre at Zhejiang University for facility support and W. Wang at the State Key Laboratory of Modern Optical Instrumentation for assistance with FIB.
Author information
Affiliations
Contributions
J.F. conceived the idea, designed and supervised all experiments, and wrote the manuscript. J.D. prepared the samples, performed the experiments and analysed the data. Y.L. performed super-resolution imaging and image analysis. Y.X. conducted data analysis and sample characterizations. F.C. prepared cell samples and performed labelling. J.Y. and Y.C. carried out experimental characterizations.
Corresponding author
Ethics declarations
Competing interests
The authors declare no competing interests.
Additional information
Peer review information Nature thanks the anonymous reviewers for their contribution to the peer review of this work.
Publisher’s note Springer Nature remains neutral with regard to jurisdictional claims in published maps and institutional affiliations.
Supplementary information
Supplementary Information
This file contains the Supplementary Methods, Supplementary Discussions, Supplementary Figs 1-31 and Supplementary References.
Video 1 Observed single-molecule ECL signals under applied voltage
Video data acquired from the EMCCD reveal the single-molecule ECL signals for the result presented in Fig. 1. Limited by the data size, only part of the raw data is shown.
Video 2 Influence of exposure time during the acquisition
With increasing exposure time, the appearance of the single-molecule ECL events changes from isolated to continuous signals (Fig. 2).
Video 3 Concentration dependence of stochastic observations
The video data correspond to results shown in Supplementary Fig. 15, which is also discussed in Fig. 3.
Video 4 The processing of super-resolved ECL imaging
The video data (for Fig. 4) reveal the processing of the single-molecule localizations of ECL reactions frame by frame. A ROI of Fig. 4 is used in the video for illustration. After localizing individual emitters, a super-resolved ECL image is reconstructed.
Video 5 Processed video from live cell single-molecule ECL imaging
The video data show the dynamic visualization of cell adhesions at different moments (36 s, 48 s, 60 s, 72 s) for two selected adhesive regions in Fig. 5.
Rights and permissions
About this article
Cite this article
Dong, J., Lu, Y., Xu, Y. et al. Direct imaging of single-molecule electrochemical reactions in solution. Nature 596, 244–249 (2021). https://ift.tt/3ABKEr4
-
Received:
-
Accepted:
-
Published:
-
Issue Date:
Comments
By submitting a comment you agree to abide by our Terms and Community Guidelines. If you find something abusive or that does not comply with our terms or guidelines please flag it as inappropriate.
"direct" - Google News
August 11, 2021 at 10:15PM
https://ift.tt/3xD0pwi
Direct imaging of single-molecule electrochemical reactions in solution - Nature.com
"direct" - Google News
https://ift.tt/2zVRL3T
https://ift.tt/2VUOqKG
Direct
Bagikan Berita Ini
0 Response to "Direct imaging of single-molecule electrochemical reactions in solution - Nature.com"
Post a Comment