Abstract
Synthetic chemistry aims to build up molecular complexity from simple feedstocks1. However, the ability to exert precise changes that manipulate the connectivity of the molecular skeleton itself remains limited, despite possessing substantial potential to expand the accessible chemical space2,3. Here we report a reaction that ‘deletes’ nitrogen from organic molecules. We show that N-pivaloyloxy-N-alkoxyamides, a subclass of anomeric amides, promote the intermolecular activation of secondary aliphatic amines to yield intramolecular carbon–carbon coupling products. Mechanistic experiments indicate that the reactions proceed via isodiazene intermediates that extrude the nitrogen atom as dinitrogen, producing short-lived diradicals that rapidly couple to form the new carbon–carbon bond. The reaction shows broad functional-group tolerance, which enables the translation of routine amine synthesis protocols into a strategy for carbon–carbon bond constructions and ring syntheses. This is highlighted by the use of this reaction in the syntheses and skeletal editing of bioactive compounds.
Access options
Subscribe to Journal
Get full journal access for 1 year
199,00 €
only 3,90 € per issue
Tax calculation will be finalised during checkout.
Rent or Buy article
Get time limited or full article access on ReadCube.
from$8.99
All prices are NET prices.
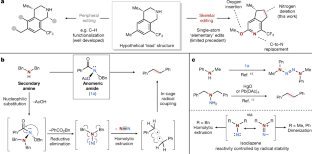
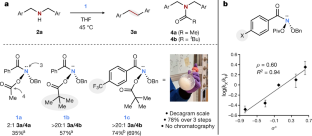
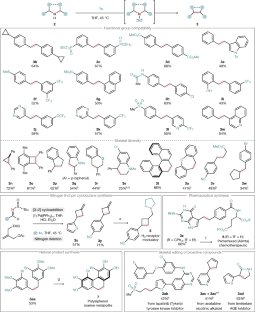
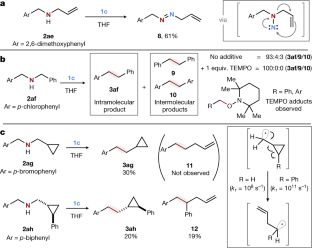
Data availability
All data are available from the corresponding author upon reasonable request.
References
- 1.
Corey, E. J. & Cheng, X.-M. The Logic of Chemical Synthesis (Wiley, 1995).
- 2.
Blakemore, D. C. et al. Organic synthesis provides opportunities to transform drug discovery. Nat. Chem. 10, 383–394 (2018).
- 3.
Huigens, R. W., III et al. A ring-distortion strategy to construct stereochemically complex and structurally diverse compounds from natural products. Nat. Chem. 5, 195–202 (2013).
- 4.
Szpilman, A. M. & Carreira, E. M. Probing the biology of natural products: molecular editing by diverted total synthesis. Angew. Chem. Int. Ed. 49, 9592–9628 (2010).
- 5.
Cernak, T., Dykstra, K. D., Tyagarajan, S., Vachal, P. & Krska, S. W. The medicinal chemist’s toolbox for late stage functionalization of drug-like molecules. Chem. Soc. Rev. 45, 546–576 (2016).
- 6.
Hu, Y., Stumpfe, D. & Bajorath, J. Recent advances in scaffold hopping. J. Med. Chem. 60, 1238–1246 (2017).
- 7.
Mahjour, B., Shen, Y., Liu, W. & Cernak, T. A map of the amine–carboxylic acid coupling system. Nature 580, 71–75 (2020).
- 8.
Cao, Z.-C. & Shi, Z.-J. Deoxygenation of ethers to form carbon–carbon bonds via nickel catalysis. J. Am. Chem. Soc. 139, 6546–6549 (2017).
- 9.
Roque, J. B., Kuroda, Y., Göttemann, L. T. & Sarpong, R. Deconstructive diversification of cyclic amines. Nature 564, 244–248 (2018).
- 10.
Smaligo, A. J. et al. Hydrodealkenylative C(sp3)–C(sp2) bond fragmentation. Science 364, 681–685 (2019).
- 11.
Fier, P. S., Kim, S. & Maloney, K. M. Reductive cleavage of secondary sulfonamides: converting terminal functional groups into versatile synthetic handles. J. Am. Chem. Soc. 141, 18416–18420 (2019).
- 12.
Xu, Y. et al. Deacylative transformations of ketones via aromatization-promoted C–C bond activation. Nature 567, 373–378 (2019).
- 13.
Silva Jr, L. F. in Stereoselective Synthesis of Drugs and Natural Products Vol. 1 (eds Andrushko, N. & Andrushko, V.) Ch. 18 (Wiley, 2013).
- 14.
Donald, J. R. & Unsworth, W. P. Ring-expansion reactions in the synthesis of macrocycles and medium-sized rings. Chem. Eur. J. 23, 8780–8799 (2017).
- 15.
Glover, S. A. Anomeric amides — Structure, properties and reactivity. Tetrahedron 54, 7229–7271 (1998).
- 16.
Glover, S. A. & Mo, G. Hindered ester formation by SN2 azidation of N-acetoxy-N-alkoxyamides and N-alkoxy-N-chloroamides—novel application of HERON rearrangements. J. Chem. Soc., Perkin Trans. 2 1728–1739 (2002).
- 17.
Hinman, R. L. & Hamm, K. L. The oxidation of 1,1-dibenzylhydrazines. J. Am. Chem. Soc. 81, 3294–3297 (1959).
- 18.
Hinsberg, W. D. & Dervan, P. B. Synthesis and direct spectroscopic observation of a 1,1-dialkyldiazene. Infrared and electronic spectrum of N-(2,2,6,6-tetramethylpiperidyl)nitrene. J. Am. Chem. Soc. 100, 1608–1610 (1978).
- 19.
Kuznetsov, M. A. & Ioffe, B. V. The present state of the chemistry of aminonitrenes and oxynitrenes. Russ. Chem. Rev. 58, 732 (1989).
- 20.
Urry, W. H., Kruse, H. W. & McBride, W. R. Novel organic reactions of the intermediate from the two-electron oxidation of 1,1-dialkyl hydrazines in acid. J. Am. Chem. Soc. 79, 6568–6569 (1957).
- 21.
Lemal, D. M. & Rave, T. W. Diazenes from Angeli’s Salt. J. Am. Chem. Soc. 87, 393–394 (1965).
- 22.
Zou, X., Zou, J., Yang, L., Li, G. & Lu, H. Thermal rearrangement of sulfamoyl azides: reactivity and mechanistic study. J. Org. Chem. 82, 4677–4688 (2017).
- 23.
Brown, D. G. & Boström, J. Analysis of past and present synthetic methodologies on medicinal chemistry: where have all the new reactions gone? J. Med. Chem. 59, 4443–4458 (2016).
- 24.
Berger, K. J. & Levin, M. D. Reframing primary alkyl amines as aliphatic building blocks. Org. Biomol. Chem. 19, 11–36 (2021).
- 25.
Plunkett, S., Basch, C. H., Santana, S. O. & Watson, M. P. Harnessing alkylpyridinium salts as electrophiles in deaminative alkyl–alkyl cross-couplings. J. Am. Chem. Soc. 141, 2257–2262 (2019).
- 26.
Brown, H. C. & Okamoto, Y. Electrophilic substituent constants. J. Am. Chem. Soc. 80, 4979–4987 (1958).
- 27.
Banert, K. et al. Steric hindrance underestimated: it is a long, long way to tri-tert-alkylamines. J. Org. Chem. 83, 5138–5148 (2018).
- 28.
Procopiou, G., Lewis, W., Harbottle, G. & Stockman, R. A. Cycloaddition of chiral tert-butanesulfinimines with trimethylenemethane. Org. Lett. 15, 2030–2033 (2013).
- 29.
Chandrasekaran, R. Y. & Wager, T. T. Histamine-3 receptor modulators. US patent US2005171181A1 (2005).
- 30.
Wager, T. T. et al. Discovery of two clinical histamine H3 receptor antagonists: trans-N-ethyl-3-fluoro-3-[3-fluoro-4-(pyrrolidinylmethyl)phenyl]cyclobutanecarboxamide (PF-03654746) and trans-3-fluoro-3-[3-fluoro-4-(pyrrolidin-1-ylmethyl)phenyl]-N-(2-methylpropyl)cyclobutanecarboxamide (PF-03654764). J. Med. Chem. 54, 7602–7620 (2011).
- 31.
Taylor, E. C. in Successful Drug Discovery (eds Fischer, J. & Rotella, D. P.) 157–180 (John Wiley & Sons, Ltd, 2015).
- 32.
Barrett, T. N., Braddock, D. C., Monta, A., Webb, M. R. & White, A. J. P. Total synthesis of the marine metabolite (±)-polysiphenol via highly regioselective intramolecular oxidative coupling. J. Nat. Prod. 74, 1980–1984 (2011).
- 33.
Moy, B., Kirkpatrick, P., Kar, S. & Goss, P. Lapatinib. Nat. Rev. Drug Discov. 6, 431–432 (2007).
- 34.
Ayer, W. A. & Habgood, T. E. in The Alkaloids: Chemistry and Physiology Vol. 11 (ed. Manske, R. H. F.) Ch. 12, 459–510 (Academic Press, 1968).
- 35.
Münch, G. et al. The cognition-enhancing drug tenilsetam is an inhibitor of protein crosslinking by advanced glycosylation. J Neural Transm. Park. Dis. Dement. Sect. 8, 193–208 (1994).
- 36.
De Almeida, M. V. et al. Preparation and thermal decomposition of N,N′-diacyl-N,N′-dialkoxyhydrazines: synthetic applications and mechanistic insights. J. Am. Chem. Soc. 117, 4870–4874 (1995).
- 37.
Glover, S. A. et al. The HERON reaction — origin, theoretical background, and prevalence. Can. J. Chem. 83, 1492–1509 (2005).
- 38.
Strick, B. F., Mundal, D. A. & Thomson, R. J. An oxidative [2,3]-sigmatropic rearrangement of allylic hydrazides. J. Am. Chem. Soc. 133, 14252–14255 (2011).
- 39.
Nwachukwu, C. I., McFadden, T. P. & Roberts, A. G. Ni-catalyzed iterative alkyl transfer from nitrogen enabled by the in situ methylation of tertiary amines. J. Org. Chem. 85, 9979–9992 (2020).
- 40.
Newcomb, M. in Encyclopedia of Radicals in Chemistry, Biology and Materials (eds Chatgilialoglu, C. & Studer, A.) (Wiley, 2012).
- 41.
Herk, L., Feld, M. & Szwarc, M. Studies of “Cage” Reactions. J. Am. Chem. Soc. 83, 2998–3005 (1961).
- 42.
Carpenter, B. K. Dynamic behavior of organic reactive intermediates. Angew. Chem. Int. Ed. 37, 3340–3350 (1998).
Acknowledgements
We thank D. Nagib, F. D. Toste, M. Johnson and Z. Wickens for discussions. Financial support for this work was provided by start-up funding from the University of Chicago.
Author information
Affiliations
Contributions
S.H.K., K.J.B. and B.D.D. designed and conducted experiments, and collected and analysed the data. M.D.L. supervised the research, conceived of the project and wrote the manuscript with input from all authors.
Corresponding author
Ethics declarations
Competing interests
Reagent 1c is under development for commercialization with Sigma-Aldrich (product number 919799), but the authors have retained no financial interest and no patents have been filed.
Additional information
Peer review information Nature thanks the anonymous reviewers for their contribution to the peer review of this work.
Publisher’s note Springer Nature remains neutral with regard to jurisdictional claims in published maps and institutional affiliations.
Supplementary information
Supplementary Information
This file contains Supplementary Sections 1-59, including Supplementary Materials and Methods, Procedures, Supplementary Figures 1-17, Supplementary References and NMR Spectra data – see contents page for details.
Rights and permissions
About this article
Cite this article
Kennedy, S.H., Dherange, B.D., Berger, K.J. et al. Skeletal editing through direct nitrogen deletion of secondary amines. Nature 593, 223–227 (2021). https://ift.tt/33G14Ay
-
Received:
-
Accepted:
-
Published:
-
Issue Date:
Comments
By submitting a comment you agree to abide by our Terms and Community Guidelines. If you find something abusive or that does not comply with our terms or guidelines please flag it as inappropriate.
"direct" - Google News
May 12, 2021 at 10:07PM
https://ift.tt/3f65m9m
Skeletal editing through direct nitrogen deletion of secondary amines - Nature.com
"direct" - Google News
https://ift.tt/2zVRL3T
https://ift.tt/2VUOqKG
Direct
Bagikan Berita Ini
0 Response to "Skeletal editing through direct nitrogen deletion of secondary amines - Nature.com"
Post a Comment